Introduction
“Essentially, all life depends upon the soil ... There can be no life without soil and no soil without life; they have evolved together.” --- Charles E. Kellogg, USDA Yearbook of Agriculture, 1938.
This is the third and final fact sheet in a series of three publications pertaining to soil properties. Part one was “Physical Characteristics of Soils, Fact Sheet, 13-02.” Part two was the “Biological Characteristics of Soils, Fact Sheet, 12-03.” This fact sheet will provide an overview of the chemical characteristics of soils.
Soil chemistry is the interaction of various chemical constituents that takes place among soil particles and in the soil solution, or the water retained by soil. The chemical interactions that occur in soil are highly complex, but understanding certain basic concepts will better help you manage your soils.
Soil pH
Soil pH is perhaps the single most important aspect of soil chemistry, because it affects the availability of nutrients to plants and the activity of microorganisms in the soil. Soil pH is a measure of the number of hydrogen ions (H+) present in a solution. In more common terms, it is a measure of alkalinity and acidity. The pH scale runs from 0 to 14. Seven is neutral, 0 is the most acidic value possible, and 14 is the most alkaline, or basic, value.
While many plants can tolerate pH ranges between 5.2 and 7.8, most plants grow best in mineral soils (soils with less than 20 percent organic content) when soil pH is between 6.0 and 7.0 (slightly acid to neutral) (Rosen, 2014).
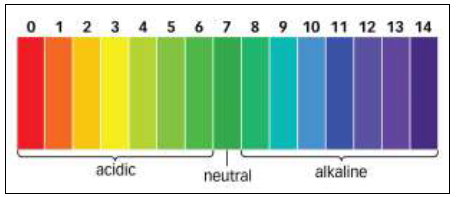
Figure 1. pH Scale.
The parent material of soils initially influences soil pH. For example, granitic soils are acidic, and limestone-based soils are alkaline. However, soil pH can change over time. Soils become acidic through natural processes as well as human activities. Rainfall and climatic conditions influence the pH of most soils. In humid climates, such as the northeastern United States, heavy rainfall percolates through the soil. When it does, it leaches basic ions, such as calcium and magnesium, and replaces them with acidic ions, such as hydrogen and aluminum. In arid regions of the country (with less than 20 inches of rain per year), soils tend to become alkaline, because salts accumulate at the surface during evaporation and rainfall is not heavy enough to leach basic ions from soils in these areas.
Human activities that increase soil acidity include fertilization with ammonium or sulfur containing fertilizers and production of industrial by-products, such as sulfur dioxide and nitric acid, which ultimately enter the soil via rainfall. Irrigating with water high in bicarbonates gradually increases soil pH and can lead to alkaline conditions.
In most cases, changes in soil pH, whether influenced by natural processes or human activities, occur slowly. This is due to the tremendous buffering capacity (resistance to change in pH) of most mineral soils. An exception to this is high-sand-content soils, where buffering tends to be low.
Nutrient availability varies markedly according to pH. This is the main reason pH is so critical for plant growth and reproduction. The optimal pH for overall nutrient availability is around 6.5 (Figure 2).
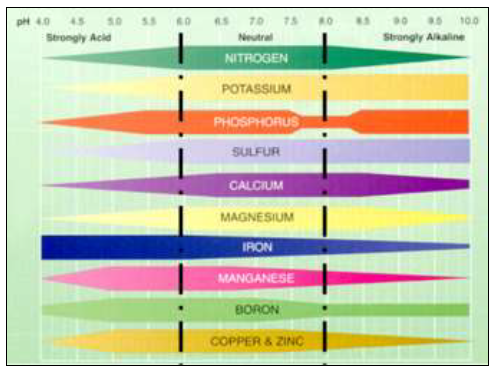
Figure 2. Nutrient availability and microbial activity as affected by soil pH. The wider the band, the greater the availability of nutrients or activity of microbes. (Adapted from Truog, USDA Yearbook of Agriculture 1943-1947)
Cation-Exchange Capacity
As previously mentioned, soil chemistry is the interaction of various chemical constituents that takes place among soil particles and in the soil solution (the water retained by soil). Soils hold onto nutritional elements similar to the way they retain water. Positively charged nutrient molecules, called cations, are attracted to the negative charges on soil particles. This is called adsorption. The sites where cations attach to particles are cationexchange sites.
The ability to hold cation nutrients is called the cation-exchange capacity (CEC). It is the ability of the soil’s negatively charged colloids to hold onto nutrients and prevent them from leaching beyond plant roots. The greater cation-exchange capacity a soil has, the more likely the soil will have a higher fertility level. When combined with other measures of soil fertility, CEC is a good indicator of soil quality and productivity (Chapman, 1965).
Clay soils retain more nutrients than coarser/sandier soils, just as clay soils hold more water, because of the greater surface area (greater number of cation-exchange sites) to which nutrients can adsorb. Organic matter also has negatively charged sites that attract and hold positively charged particles. Thus, sandy soils rely on organic matter content to increase cation-exchange capacity.
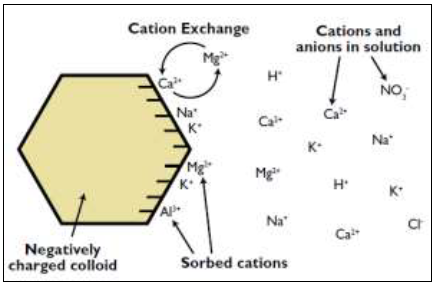
Figure 3. Representation of exchange capacity, (Adapted from Brady and Weil, 2002)
Thus, CEC is important for maintaining adequate quantities of plant-available calcium (Ca2+), magnesium (Mg2+) and potassium (K+), among other cations found in the soil (Figure 3). Cation-exchange capacity can be expressed two ways:
- the number of cation adsorption sites per unit weight of soil
- the sum total of exchangeable cations a soil can adsorb.
Soil CEC is normally expressed in units of charge per weight of soil. Two different, but numerically equivalent sets of units are used: meq/100 g (milliequivalents of element per 100 grams of dry soil) or cmolc/kg (centimoles of charge per kilogram of dry soil).
The unit of milliequivalents (meq) per 100 grams of oven-dry soil is used to better reflect that it is the “charge” in the soil that determines how many cations can be attracted.
Salt-Affected Soils
The presence and concentration of salts in soil can have adverse effects on soil function and management. Salt-affected soils are most common in arid and semiarid regions, where evaporation exceeds precipitation and dissolved salts are left behind to accumulate, or in areas where vegetation or irrigation changes have caused salts to leach and accumulate in low-lying places (saline seeps). The three main types of salt-affected soils are saline, sodic and saline-sodic.
Saline soils contain a high level of soluble salts in the soil-water solution. Most common salts are sulfates of sodium, calcium, magnesium and potassium (depending on the soil region). The primary effect of excessive soluble salts on plants is to limit the ability of plant roots to absorb soil water, even under wet soil conditions. Soil water flows from higher osmotic potential (low-salt concentration) to lower osmotic potential (high-salt concentration). A soil solution with low osmotic potential due to the higher concentration of soluble salts compared to plant cells, will not allow plant roots to extract water from soil, causing drought-like symptoms in the plants (Seelig, 2000). As the word “soluble” indicates, these salts can easily be leached out of the upper 2 to 3 feet of surface soil under good soil moisture and drainage conditions.
Sodic soils have excessive levels of sodium adsorbed at the cation exchange sites. Soil sodicity causes degradation of soil structure. That process is called soil dispersion. The forces that hold clay particles together are greatly weakened when excessive sodium is adsorbed at the negative charges of clay particles, forming sodium-clay particles (Seelig, 2000).
Whereas, wet, sodium-clay particles get easily disintegrated or dispersed from the larger soil aggregates, once dry, sodiumclay particles clog the soil pores (especially macro-pores) and settle down in dense layers. The resulting poor physical structure then results in soils difficult to till, poor seed germination and restricted plant root growth.
Saline-sodic soils have both high soluable salt and sodium content. However, if existing soluble salts are leached downward while exchangeable sodium in the soil profile remains constant, soil properties are likely to closely resemble those of sodic soils.
Summary
Chemical properties of the soil are important to soil fertility, plant growth and reproduction. That is why it is important to understand how chemical properties of the soil interact to affect the soil’s capacity to store and release nutrients, and how soil chemistry can affect soil structure.
Soil chemisty is a very complex subject. Due to the brevity of this publication only the basic principles have been discussed. For a more comprehensive look at soil properties and health information, go to the United States Department of Agriculture, Natural Resources Conservation Service’s “Soil Health” website located at: United States Department of Agriculture.
Work Cited
Brady, N. and R. Weil. 2002. The Nature and Properties of Soils, 13th Edition. Prentice Hall. Upper Saddle River, New Jersey. 960 p.
Chapman, H.D.1965. Cation-exchange capacity. Methods of soil analysis - Chemical and microbiological properties. Agronomy 9: 891-901.
Kellogg, C. E.1938. Soil and Society, United States Department of Agriculture, Yearbook of Agriculture, pg. 863.
Rosen, C.J., P.M. Bierman, R.D. Eliason, 2014. Nutrient Cycling and Maintain Soil Fertility in Fruit and Vegetable Crop Systems, Department of Soil, Water, and Climate. University of Minnesota.
Seelig, B.D. 2000. Salinity and Sodicity in North Dakota Soils. EB-57. North Dakota State University, Fargo, ND.
Truog, E., 1943-1947. The Liming of Soils, United States Department of Agriculture, Yearbook of Agriculture, pg. 566.