What are high tunnels and why do growers use them?
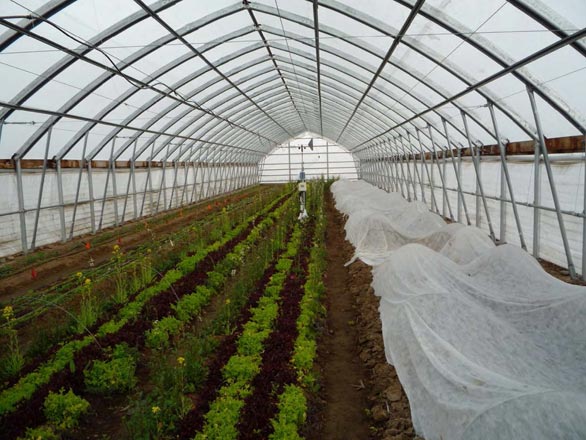
High tunnels, like that shown above, are single or multi-bay greenhouse-like structures, usually unheated and covered by one or two layers of greenhouse plastic film, in which temperature and humidity are managed by some combination of roll-up side vents, end-wall vents and fans. The type of structure varies by location, based on the farmers’ needs and local climate concerns, such as wind, rain and snow load (Blomgren and Frisch, 2007; Lamont et al., 2002). In the United States, high tunnel adoption has increased as local food movements across the country have led to greater demand for fresh, local produce (Carey et al., 2009). In general, high tunnels are used for growing high-value specialty crops, such as berries, tree fruit, cut flowers and a wide variety of vegetables (Lamont, 2009). Although research results and educational resources about growing crops in high tunnels are available (Carey et al., 2009), additional research and education will be necessary to address information gaps on labor management, soil and climate (Carey et al., 2009; Montri and Biernbaum, 2009; Fitzgerald and Hutton, 2012) as high tunnels grow in popularity.
One of the main proposed benefits of high tunnels is the ability to manipulate the microclimate, the climate conditions immediately around the plants, to improve crop growth. Because most high tunnels use passive heating and cooling, they are highly influenced by local climatic conditions and management practices. In particular, high tunnels have grown in popularity in places with short and variable growing seasons, such as high deserts. Most high tunnel research has focused on crop growth and yield, so little information is available comparing microclimates inside and outside high tunnels, especially in high-desert regions.
Research in other environments
Although relatively few researchers have undertaken comprehensive assessments designed to compare multiple aspects of the microclimates inside and outside of high tunnels, numerous studies tracking crop growth or insect, pest and pathogen activity measured some aspects of microclimate. All of the studies reviewed showed that high tunnels reduce solar radiation (Borrelli et al., 2013; Both et al., 2007; Bumgarner et al., 2012; Cowan et al., 2014; Lang, 2009; Lang 2014; Lang et al. 2011; Olberg and Lopez, 2016; Owen et al., 2016; Reiss et al., 2004; Rom et al., 2010; Thompson et al., 2009; Zhao and Carey, 2009) and wind speed (Cowan et al., 2014; Lang, 2009; Wallace et al., 2012; Zhao and Carey, 2009) within the high tunnel relative to outside of the high tunnel.
Despite reductions in the amount of solar radiation, most studies have documented higher maximum temperatures inside high tunnels than outside (Cowan et al., 2014; Gent, 2002; Gu et al., 2017; Kadir et al., 2006; Lang, 2014; O’Connell et al., 2012; Ogden and van Iersel, 2009; Ogden et al., 2011; Rader and Karlsson, 2006; Rogers and Wszelaki, 2012; Rogers et al., 2016; Shiwakoti et al., 2016; Vescera and Brown, 2016; Wallace et al., 2012; Zhao and Carey, 2009; Zhao et al., 2014). In the few cases where maximum or daytime temperatures were not higher in high tunnels than surrounding areas, it was typically because the high tunnel was covered with shade cloth (Rowley et al., 2011; Zhao and Carey, 2009). Two studies focused on winter or spring, however, found conditions within high tunnels to be similar (Santos and Salame-Donoso, 2012) or even cooler (Wallace et al., 2012) than outside.
The effect of high tunnels on minimum temperatures was not as clear. A number of studies documented higher minimum temperatures inside than outside of high tunnels, although many of those increases were relatively modest (Both et al., 2007; Cowan et al., 2014; Gent, 2002; Gu et al., 2017; Kadir et al., 2006; Martin and Sideman, 2012; O’Connell et al., 2012; Rader and Karlsson, 2006; Rowley et al., 2014; Santos and Salame-Donoso, 2012; Santos et al., 2014; Shiwakoti et al., 2016; Ward and Bomford, 2013; Zhao and Carey, 2009; Zhao et al., 2014). A substantial minority of studies, however, found that temperatures inside high tunnelss were similar to or even cooler than outside of the structures (Ogden and van Iersel, 2009; Ogden et al., 2011; Rogers and Wszelaki, 2012; Rogers et al., 2016; Vescera and Brown, 2016; Vescera and Brown 2011; Wallace et al., 2012).
Although there has been extensive work on the topic of high tunnel microclimates, little research has targeted the use of high tunnels in high- or cold-desert environments. Moreover, microclimatic monitoring in those environments appears to be accessory to production evaluations in the few available studies. For example, Rowley et al. (2011) measured air temperature as part of a study evaluating strawberry success inside and outside of high tunnels in northern Utah. Likewise, Shiwakoti et al. (2016) monitored high tunnel air temperature as part of a study focused on the yield and quality of several common kitchen herbs in Wyoming. Winter air temperatures inside and outside of high tunnels in Idaho and eastern Washington were presented as supporting information for research focused on the growth of salad and cooking greens (Borrelli et al., 2013). Wallace et al. (2012) conducted a study on lettuce production using high tunnels located in Lubbock, Texas, which is arid, but substantially warmer than western Nevada. Consequently, this is one of the first studies documenting the results of comprehensive microclimate monitoring within high tunnels in the Intermountain West. The objective of our study was to examine temperature, vapor pressure deficit (VPD), wind speed and solar radiation inside and outside high tunnels in the Great Basin region of western Nevada to provide baseline information about the impact of high tunnels on microclimate in the high desert.
Research design
We conducted this work at the Desert Farming Initiative (DFI, 39.5384°N, 119.8049°W, elevation 4493 ft.) in the University of Nevada, Reno Experiment Station on Valley Road in Reno, Nevada from March 2016 to April 2017. We used existing gothic-style high tunnels (FarmTek GrowSpan Gothic Premium High Tunnels, Dyersville, Iowa) constructed from triple-galvanized structural steel tubing with a 0.3 mm woven polyethylene fabric covering (PolyMax® 5.2 oz.,12 mil Clear Woven Greenhouse Covering, 85% light transmission when new) and manual roll-up sides. Onset® weather stations (U30NRC, Bourne, Massachusetts) were installed in two 26-foot by 124-foot high tunnels oriented east-west, two 26-foot by 96-foot high tunnels oriented north-south, and in a nearby outside plot. Crops growing inside the high tunnels at various times during the data collection period were leaf lettuce, carrot, radish, bell pepper and tomato. The farm manager determined fertilizer plans, irrigation scheduling, pest control and planting schedules.
Inside, weather stations were placed in the center crop bed, 12.5 m and 9.8 m from the high tunnel entrances (Figures 1 and 2). Outside, one weather station was located in the center crop bed in the center of the plot (Figure 2). Each weather station had a temperature and relative humidity sensor covered in a solar radiation shield (S-THB-M002, RS3, Bourne, Massachusetts) positioned at 1 m and 16 cm above the soil surface to account for microclimates of crops of differing heights. The sensor recorded temperature with an accuracy of ± 0.2 degrees C and a measurement resolution of 0.02 degrees C between -40 degrees C and 75 degrees C and humidity with an accuracy of ± 2.5% and measurement resolution of 0.1% between 10 and 90%, with accuracies closer to 5% under very dry and very humid conditions. A silicon pyranometer (S-LIB-M003, M-LBB, M-LLA, Bourne, Massachusetts) recorded incoming solar radiation with an accuracy of ± 10 W/m2. An anemometer (S-WSET-B, M- CAA, Bourne, Massachusetts) measured wind speed with an accuracy of ± 1.1 m/s at approximately 1.5 m. Weather stations recorded measurements every 15 minutes.
Sensors were tested for precision by deploying them for 24 hours in a controlled setting to confirm that they produced similar measurements before they were installed in the field. Data collected on October 10, 2016 in one of the east-west high tunnels were removed from the study because supplemental heating was brought in for a farm-to-table dinner. One of the east-west high tunnels only had data from January 2017 to April 2017 because the high tunnel cover blew off and sensors malfunctioned. For data from all sensors, measurements made every 15 minutes were averaged to hourly. Daily maxima and minima were isolated from the hourly averaged data for each complete 24-hour period starting at midnight Pacific Daylight Time (PDT). The diurnal temperature range was calculated using the daily maximum and minimum temperatures. Relative humidity was converted to vapor pressure, and saturation vapor pressure was calculated from temperature using the Clausius-Clapeyron equation in the humidity package (Cai, 2016) in R (R Core Team, 2017). Vapor pressure was subtracted from saturation vapor pressure to calculate the vapor pressure deficit (Abtew and Melesse, 2013). Because of the small numbers of replicates (one weather station outside of the high tunnels and only one or two measurements within each high tunnel), it was not possible to conduct any robust statistical analyses. Making additional measurements to provide more replication was not feasible due to cost limitations and the desire to minimize impacts on operations at Desert Farming Initiative.
Reduced solar radiation inside high tunnels
Solar radiation inside all high tunnels was reduced relative to outside in all seasons. This was expected, as even new, the high tunnel cover allows only 85% light transmission, and the cover likely had been in place for three to four years. Moreover, the amount of reduction varied depending on the orientation of the high tunnel and the time of year (Figure 3). Annually, the north-south high tunnels experienced greater reductions in solar radiation than the east-west high tunnels. Early in the season, maximum solar radiation rose quickly inside the high tunnels, similar to trends outside the high tunnel. Solar radiation in our high tunnels leveled off from May to July, irrespective of high tunnel orientation, before decreasing in August. It is possible that the summertime plateau in radiation is related to the style of high tunnels at Desert Farming Initiative. The amount of incoming solar radiation that the high tunnel cover transmits varies with the angle of the sun relative to the angle of the high tunnel roof. While Quonset-style high tunnels generally transmit the most solar radiation in the summer, when the sun is highest in the sky (Blomgren and Frisch, 2007), gothic-style high tunnels, such as those at Desert Farming Initiative, receive the most solar radiation in the spring and fall when the angle of the incoming solar radiation is more perpendicular to the angle of the high tunnel roof. This effect is often increased in east-west oriented gothic-style high tunnels (Blomgren and Frisch, 2007), as we observed at Desert Farming Initiative.
Regardless of high tunnel orientation, however, the light transmitted through the high tunnel cover was adequate to meet plant photosynthesis requirements during the growing season (Figure 3). The light saturation point, indicated by the dashed line on the graph, shows the point at which an increase in solar radiation above the line does not result in an increased plant photosynthetic rate, assuming adequate CO2 and optimal temperatures. Below about 200 W/m2, lack of light may limit plant growth. During this time (December and January), high tunnel production could focus on plant propagation by seeds or cuttings.
To read the complete paper on using high tunnels in Nevada, use the following link to download the PDF version of the document.