Introduction
Low Impact Development (LID) is a sustainable approach to managing stormwater on developed sites using design techniques that detain, retain, infiltrate, filter and evaporate runoff close to its source. When stormwater infiltration occurs close to the source, the likelihood of soil erosion and increased pollutants in the runoff decreases.
The original hydrology of the site, the ability of the site to infiltrate stormwater, and the amount of stormwater that runs off an undeveloped site are dependent on the type of soil present. Site-specific soil properties thus influence the selection of the best method of stormwater treatment for developed sites, as well as the cost.
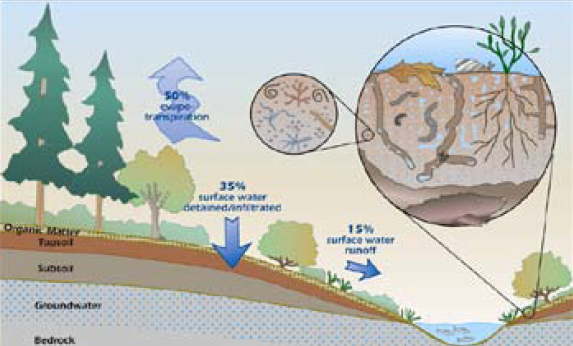
Stormwater distribution with ample soil biota (above) is shown on a natural or undeveloped site, while stormwater distribution and depleted soil biota (below) are shown on a developed site. Images by Washington Organic Recycling Council.
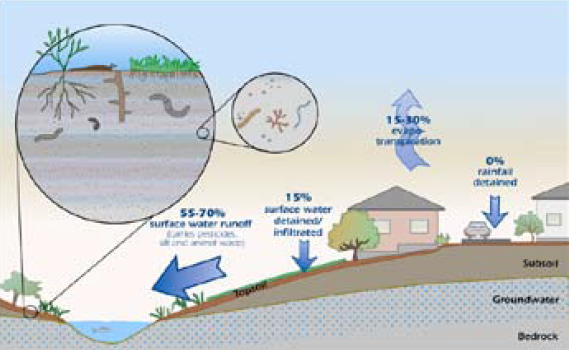
Benefits of Good Soil:
- INFILTRATES stormwater
- PROCESSES pollutants
- HOLDS water and nutrients for plants
- PROVIDES pore space for biological activity
How does development affect the amount of stormwater infiltrated into soils?
When rain or snow falls on undisturbed, vegetated soil, about half the precipitation is lost to evapotranspiration, which is the combination of transpiration by plants and direct evaporation (see graphic ). Of the other half of the precipitation, about 35 percent soaks (infiltrates) into the soil profile and 15 percent runs off. After development occurs, and impervious surfaces such as pavement or buildings cover the soil, this ratio changes dramatically. As much as 55 to 70 percent runs off the site, and as little as 15 percent or less infiltrates.
How does soil compaction affect LID?
While some increase in pore space occurs naturally through freeze-thaw cycles and other mechanisms, most soil compaction is not easily reversed. The net result is an increase in the amount of runoff from the site, less water storage, less biological processing and more soil erosion. Compacted soils are not appropriate for LID.
How are soil properties determined?
The type of native soil that forms at a site is influenced by the original type of rock present on the site; how long weathering has occurred; the local climate; land surface features or topography, including slope; and the actions of plants and animals. These factors influence the chemical, physical and biological properties of a soil. The soil at the surface, called the topsoil, is generally highest in organic matter and most valuable for landscaping.
Determining the best LID practice or practices to implement on a site-by-site basis requires a comprehensive inventory of site conditions. Start by performing an assessment of onsite soil properties and site hydrology, including soil textural analyses and onsite infiltration measurements.
Collect and submit a soil sample for analysis to determine texture, nutrient levels and the type of clay present. If there are significantly different types of soil on the site, collect separate samples from the different areas for analysis.
Maps providing very general information on surface soils in the greater Truckee Meadows area are provided in Appendix A of the Truckee Meadows LID Handbook. The maps provide very general information on surface soils of the area, and should not be substituted for an onsite assessment of soil properties.
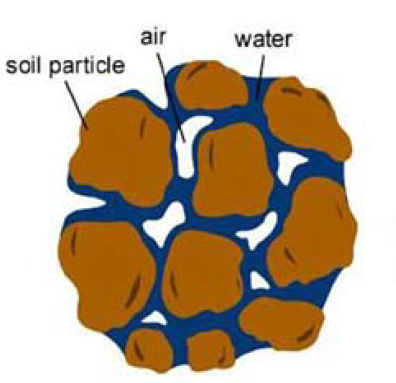
This soil has about 50 percent pore space. About half of the pore space is filled with air and half with water. Image by University of Nevada Cooperative Extension.
Soil properties: texture, porosity and infiltration
A typical soil in good condition contains about 50 to 60 percent solid material and 40 to 50 percent pore space, or gaps between the particles. In this typical soil, about half of the pore space is filled with water and half with air. The solid materials consist mainly of minerals, and include a small amount of organic material. In Nevada, the organic matter fraction tends to be very small, often under 1 percent. In contrast, in a productive agricultural soil, organic matter may range up to 5 percent. Organic matter helps increase the ability of a soil to absorb and store water and nutrients.
Soil minerals fall into three main groupings, called sand, silt and clay, based on the size of the particles. Sand grains are the largest particles (0.05 to 2 millimeters (mm) in diameter), silt grains are intermediate in size (0.002 to 0.05 mm), and clay grains are the smallest (less than 0.002 mm in diameter).
The proportion of sand, silt and clay, called soil texture, affects the porosity and permeability of the soil. Porosity is a measure of the amount of pore space in a soil, and permeability is a measure of how well-connected the pore spaces are to one another. Porosity and permeability affect infiltration rates, or how quickly water is absorbed into the soil. They also affect water-holding capacity, which influences how slowly water drains from the soil. In general, the higher the percentage of silt- and clay-sized particles, the higher the water-holding capacity.
Some Nevada locations have clay-rich soils. Infiltration into these soils is limited by the small grain size of the clay particles and the small pore spaces that remain between the clay grains. Some clay soils swell up when wetted, further reducing the pore space. The ability of clay particles to attract and hold onto water molecules makes the water-holding capacity of these soils high.
Use simple percolation tests to determine infiltration rates. For small-scale projects, dig a pit 1 to 2 feet deep. Fill the pit with water and let it soak in. Then, place a yardstick vertically in the hole and fill the pit again to a measured depth, such as 12 inches. Monitor the rate at which the water level drops and determine the infiltration rate in inches per hour. For larger-scale projects, refer to the infiltration testing methods in the Truckee Meadows Low Impact Development Handbook (www.TMstormwater.com).
LID practices are most effective in soils with infiltration rates of at least one-half inch per hour. Rates of 1 inch per hour are preferred. Amend soils with infiltration rates less than one-half inch per hour with organic material to increase their infiltration rate, or install underdrains to facilitate drainage. The table shows average infiltration rates for different soil types. Sand, loamy sand, sandy loam and loam soil types have infiltration rates appropriate for LID. All other soil types will require soil amendment to increase infiltration rates if they are to be used for LID.
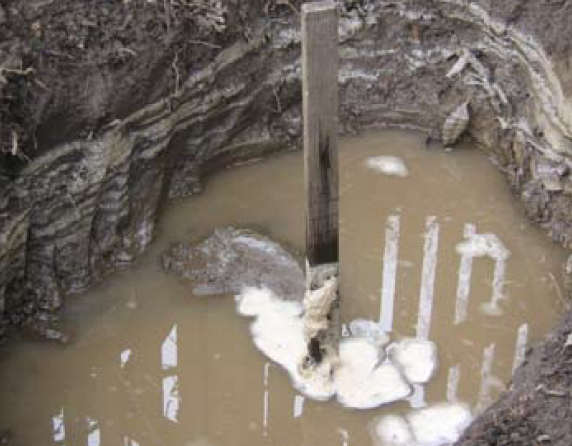
Use a ruler to measure the rate of infiltration in a pit. Image by IPMopedia.
Infiltration Rates by Soil Type Under Saturated Conditions
Soil Type |
Infiltration Rates (inches/hour) |
Infiltration Rates (inches/day) |
Sand |
8.27 |
198.5 |
Loamy Sand |
2.40 |
57.6 |
Sandy Loam |
1.02 |
24.5 |
Loam |
.52 |
12.46 |
Silt Loam |
.27 |
6.5 |
Sandy Clay Loam |
.17 |
4.1 |
Silt |
.10 |
2.4 |
Clay Loam |
.09 |
2.2 |
Silty Clay Loam |
.06 |
1.4 |
Sandy Clay |
.05 |
1.2 |
Silty Clay |
.04 |
1.0 |
Clay |
.02 |
.5 |
Source: The RETC Code for Quantifying the Hydraulic Factors of Unsaturated Soils, van Genuchten, et al., 1992
Soil biology
Air-filled pore space is essential to healthy plants and soil organisms. Soils are full of living creatures, such as earthworms, mites and other arthropods, nematodes, and microscopic plants and animals, including bacteria and fungi. Together, the living creatures are called soil biota. The soil biota population increases as the amount of organic material in the soil increases.
Soil biology plays an important role in infiltration, water-holding capacity, pollutant processing, nutrient retention, nutrient cycling and plant growth. The soil biota transform and cycle carbon, nitrogen and other mineral nutrients in plant and animal residues into forms that can be used by plants. Soil bacteria Soil biology plays an important role in infilhave a wide range of abilities to break down pollutants into less harmful components, purifying water as it moves through the soil. Soil compaction reduces pore space. This may affect the habitat available for soil biota. As the number of living organisms in the soil decreases, soil fertility also decreases, limiting plant growth.
LID is used because it is:
- EFFECTIVE in reducing and treating urban runoff
- ECONOMICAL, when less infrastructure is required for LID practices than for traditional stormwater features
- SIMPLE! Most LID features are not highly engineered, expensive or elaborate
- FLEXIBLE and customized for each site
- USEFUL in achieving multiple objectives: stormwater management, landscape beautification, habitat improvement, open space connectivity, etc.
- DESIRABLE, because it adds value when the landscape features used to achieve LID goals are also aesthetically pleasing. This can increase property values.
For a description of various Low Impact Development practices:
consult the following Fact Sheets in the “Low Impact Development in Northern Nevada” series:
- LID: An Introduction, FS-09-22
- Soil Considerations, FS-09-23
- Rainwater Harvesting, FS-09-24
- Bioretention, FS-09-25
- Vegetated Swales and Buffers, FS-09-26
- Green Roofs, FS-09-27
- Plant Materials, FS-09-28
- Porous Pavement, FS-09-29
- Roadway and Parking Lot Design, FS-09-30
- Maintenance, FS-09-31
Soil compaction
During construction and development, soil is compacted by heavy equipment and vehicular traffic. The effect increases when these disturbances occur on wet soils. Compaction results in a decrease in soil porosity, infiltration rates and the amount of water and oxygen available for plant growth and soil biota. While some increase in pore space occurs naturally through freeze-thaw cycles and other mechanisms, most soil compaction is not easily reversed. The net result is an increase in the amount of runoff from the site, less water storage, less biological processing and more soil erosion. Compacted soils are not appropriate for LID.
Construction cut and fill practices also result in soil compaction and removal of valuable topsoil. Typically, topsoil is removed, mixed with subsoils and stockpiled prior to excavation for foundations or other earthworks. The hydrology of the site is immediately changed, because topsoil and vegetation are no longer in place to infiltrate, retain or intercept precipitation and hold the soils in place. Rain falling on the site is now much more likely to run off, cause erosion and transport eroded soil particles, other debris and pollutants from the site, unless construction site best management practices (BMPs) have been installed and maintained. To make matters worse, as construction proceeds, heavy equipment further compacts the remaining soil and subsoil.
Once construction activities have been completed, typically a thin layer of imported topsoil is laid down on top of the compacted soil or subsoil and the area is landscaped. Not surprisingly, turf and other ornamental plants installed in these conditions need more water and nutrients to survive, since the compacted subsurface soils restrict root growth and soil moisture retention. Trees planted in holes dug on these sites respond as if they had been planted in plastic pots, with the trees sometimes drowning in their own irrigation water due to lack of drainage through the compacted subsoil. These trees often are unable to maintain a stable root system and may blow over during storms. Landscapes planted in compacted soils often struggle along, requiring extra care and additional inputs of fertilizers or pesticides, before they finally die due to disease and environmental stresses.
Improving compacted soils
The best solution is to avoid soil compaction whenever possible. Prior to construction, assess the site to identify the soils with the highest infiltration capacity. Design developments to protect these soils while placing any impervious surfaces, such as buildings and driveways, on the soils that have lower infiltration rates. Stockpile and replace high-quality topsoil after construction has concluded.
During construction, design and use specified travel paths to limit overall compaction of the site. Restrict heavy equipment to those areas that have soils with the lowest infiltration rates. Protect areas with good infiltration rates, including sandy and loamy soils, to preserve existing infiltration rates and healthy soil biota. Limit removal of vegetation to only what is essential for construction, to reduce the potential for compaction and erosion and preserve the original site hydrology. Flag or fence around existing vegetation that is to be preserved.
After construction, restore compacted soils by ripping and adding organic matter. Any activity on soil should occur when the soil is moist enough that digging will not create dust and dry enough that equipment driving over it does not create ruts. The most common method to reduce compaction is to rip the soil to a depth of 12 inches. In Nevada, call 1-800-227-2600, “Call Before You Dig,” prior to ripping to locate all underground gas, water, sewer, septic and utilities. Avoid these areas.
Ripping will provide a temporary increase in soil porosity, but the pore space will decrease over time. To extend the benefits, add organic material to the soil after ripping. Avoid ripping within the drip lines of existing trees to avoid damage to tree roots.
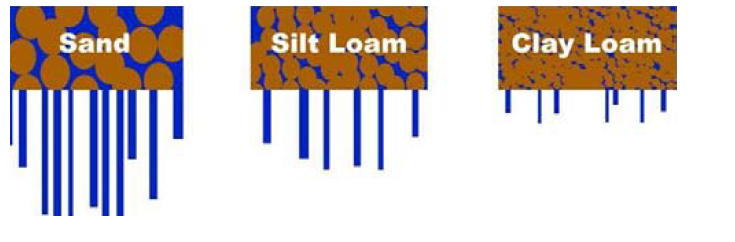
Water moves rapidly through sandy soils, and slowly through clay soils.
Adding organic matter to improve soils
Nevada soils are generally deficient in organic matter, and compacted soils will especially benefit from the addition of organic material. Compost, a mixture of decaying organic matter, such as leaves and manure, is often used as a source of organic matter. Amending with compost improves soil structure and water-holding capacity; increases onsite stormwater retention, filtration, pollutant retention, and nutrient-holding capacity; and reduces irrigation water and fertilizer requirements.
When adding organic material or compost to soil, mix one part of compost to two parts of soil. Start by loosening the existing topsoil to a depth of 8 inches. Place 4 inches of compost on the soil and mix or till it. The total depth will then be about 12 inches.
When tilling, use two passes, with the second tilling perpendicular to the first. If enough soil exists, till to a greater depth.
Use caution when selecting the size of the tilling equipment, as heavy tilling equipment will compact the soil further.
On sites with restricted access, buried utilities, or thick vegetation, the addition of 3 to 4 inches of organic mulch on the soil surface will help decrease compaction in the underlying soil with time. However, the process is much slower than direct mixing.
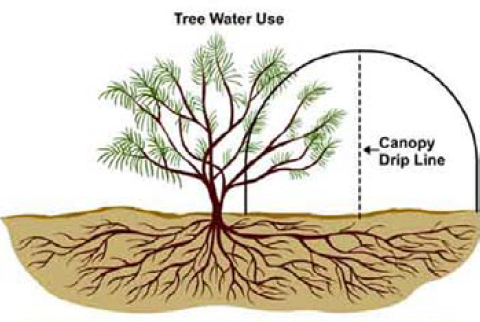
Tree roots can extend 1 1/2 to 4 times beyond the Canopy. Image by Southern Nevada Water Authority.
What’s the right soil?
Specific LID practices have their own soil requirements. For example, never use uncomposted manure as part of the engineered soil mix of LID practices such as bioretention systems, including landscape detention features, rain gardens, tree box filters and stormwater planters. These systems are designed to capture, concentrate, filter and infiltrate stormwater through engineered soils.
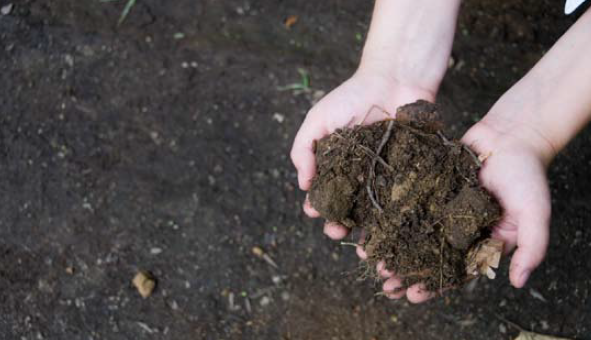
Uncomposted manure typically contains elevated levels of ammonia, phosphorus, salts and pathogens, which can leak out and harm a receiving water body. See the other fact sheets in this series for specific recommendations for each LID practice. Whenever possible, determine soil texture, organic matter content and infiltration rate of the native soil present on the site prior to selecting an LID practice.
Adding 3-4 inches of organic mulch will help decrease soil compaction and make the soil more capable of filtering stormwater. Image by CSREES.
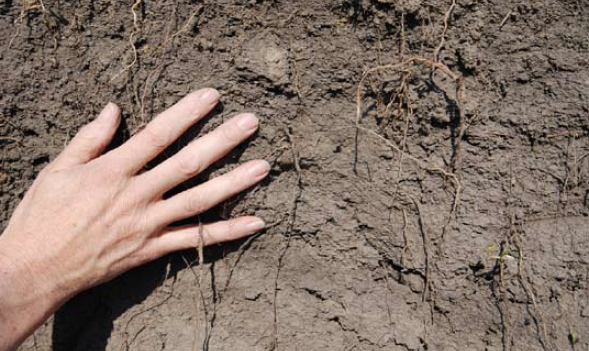
Determine soil texture before applying an LID practice to a site. Image by CSREES.
For additional detailed planning and design information
refer to the latest versions of the Truckee Meadows Low Impact Development Handbook and the Truckee Meadows Structural Controls Design Manual available at tmstormwater.
For more information about LID, see:
- Low Impact Development Center, Inc., www. LIDC.
- National Institute of Building Sciences Whole Building Design Guide, Low Impact Development Technologies, WBDG.
- Urban Design Tools, Low Impact Development, LID.
- U.S. Environmental Protection Agency Polluted Runoff (Nonpoint Source Pollution), Low Impact Development (LID), EPA.
Reference
Van Genuchten, M. Th., Leiji, F.J. and Yates, S.R., 1992, The RETC Code for the Hydraulic Functions of Unsaturated Soils, U.S. Environmental Protection Agency Research and Development, EPA/600/S2-91-065.
Technical review provided by Chris Conway, Certified Professional in Sediment and Erosion Control.