Introduction
This fact sheet is the first in a series of three publications that provide a brief overview of the physical, biological and chemical characteristics of soils. The information is provided for agronomic producers to help them understand soil properties and characteristics. Hyperlinks for words or term definitions are available for readers.
The U.S.D.A. Soil Survey Manual defines soil as “the collection of natural bodies in the earth’s [sic] surface, in places modified or even made by man of earthy materials, containing living matter and supporting or capable of supporting plants out-of-doors” (Soil Conservation Service, 1993). The American Heritage Science Dictionary defines land as ground or soil (Houghton Mifflin Company, 2005). Land, or soil, is an essential natural resource to mankind, being the literal foundation upon which life on earth is based.
Soil is a dynamic and very complex system composed of living and dead organisms, decaying and residual organic matter, minerals, air and water. The various physical, biological and chemical processes taking place in soil are difficult to understand; however, understanding soil properties helps farmers make prudent management decisions (Schulte, et al., 2005).
Soil and Land Classification
Soil names are a combination of the soil series and soil type (Soil Conservation Service, 1993). The soil series is a geographical name from the area the soil was first described and mapped.
The soil type is the textural class (e.g. loam, silty clay, etc.). The same soil series can occur across large geographic areas, including multiple counties and states.
Soils are classified in a manner similar to plants and animals. The lowest or most specific unit of soil classification is the soil series. Higher levels of classification include the family, subgroup, great group, suborder and order.
Land is classified by the United States Department of Agriculture (USDA) on the basis of permanent limitations or hazards that limit its use and sustainability. All of the soil’s properties are considered when determining the land capability class. Each characteristic is evaluated for limitations or hazards such as erosion, droughtiness, wetness, stoniness and response to management. The specific characteristics that most limit the sustainable use of a soil determine the land capability class.
There are eight recognized capability classes of land, equally divided between soils that can and cannot be cultivated. Soils in cultivable classes I to IV may be used for cultivated crops, pasture, woodland, range or wildlife food and cover. The larger the land capability class number, the more severe the limitations. Land Classifications V- VIII are considered non-cultivable and are generally not suited to tillage and cultivated crops, although Class V soils are often suited to pastures that can be improved and benefit from proper pasture management practices.
The Natural Resources Conservation Service (NRCS) provides detailed maps of soils and suggested soil and crop management practices. NRCS publishes these soil maps and detailed reports for each county. This information is available through the (NRCS) Internet Web Soil Survey, Web Soil Survey, or in printed form at local NRCS offices.
Soil Formation
Soil development is a result of the interactions of climate, vegetation and other organisms on existing geologic materials on differing topography over a given period. For the most part, soils are the same wherever all elements of the five formation factors parent material, climate, topography, biological factors and time) are the same. Under similar environments in different places, soils are similar. This regularity permits prediction of the location of many different kinds of soil (Soil Conservation Service, 1993).
Most soils in the United States have fairly definite layers or soil horizons. Each horizon has a distinct relationship with the horizon located immediately above and/or below. These relationships result from the soil-forming processes operating on the undisturbed soil material. By examining these distinguishable horizons it is possible to make recommendations for adapted crops and soil- management practices that are suited to the soil (Soil Conservation Service, 1993).
There are some minor land forms that do not exhibit these systematic horizons. The soil forming processes that produce uniform horizons have been, or are being completely disrupted by some modifying activity, such as irrigation. In Nevada, about 90 percent of the irrigated soils do not exhibit horizons developed by soil-forming processes (McCormick & Naphan, 1955), but reflect decades of supplemental irrigation. Rcommendations for soil and water management, therefore, should be based upon independent investigations (ground-truthing) of each valley, each farm and even each field, if possible, and not solely on NRCS soil surveys.
Soil Horizons
Many kinds of minerals occur in soils as naturally occurring inorganic substances. Some minerals contribute little to plant growth, while others provide many required plant nutrients. Most soils typically have a series of horizontal layers, called horizons, with varying degrees of development and thickness. The layers typically parallel the soil surface, and collectively compose the soil profile (Figure 1). A soil usually has three major horizons: (1) the topsoil or “A” horizon, (2) the subsoil or “B” horizon, and (3) the parent material or “C” horizon (Figure 1). In addition, a soil may have a leached horizon (E) or a nonmineral organic matter horizon (O), but these horizons are not common in Nevada soils (Schulte, et al., 2005).
The “A” horizon is the mineral horizon subject to the direct influences of the earth’s surface environment. It usually contains the most organic matter. Organic matter provides the living environment for important soil organisms, is the primary source of many nutrients essential for plant growth, and is an important contributor to good soil structure, which increases water storage and movement.
The “B” horizon is where clay and minerals usually accumulate.
The “C” horizon is composed of geologic materials that have not been changed appreciably by weathering processes. Parent materials are the original geologically deposited minerals from which the soil developed. The physical characteristics of each soil horizon in the profile influence the soil’s ability to retain and transmit water, affecting the crop’s root growth, water use and productivity.
Figure 1. A simplified soil profile.
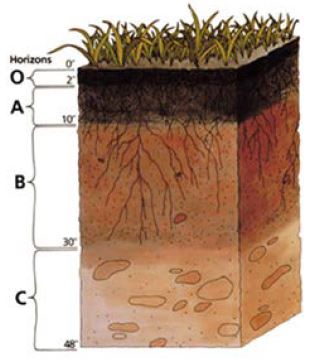
Soil Properties
Every soil has properties that fall into three distinct categories:
- Physical
- Biological
- Chemical
All of a soil’s properties, collectively, affect every producer’s decisions about how to manage the land and how the land responds to specific management practices. These properties all interact to determine the soil’s productivity.
Soil Texture
Soil texture affects physical and chemical properties that influence crop growth. In the broadest sense, soil texture refers to the “feel” of the soil, its coarseness or fineness. More specifically, it is the soil’s relative proportions of sand, silt and clay. A soil containing equal percentages of sand, silt and clay is classified as a loam (the ideal soil). In the field, soil texture can be determined by feeling the soil with the fingers (Figure 2). The USDA Soil Survey Manual includes the following general definitions of soil textural classes in terms of visually examining the soil in the field:
Figure 2. Determining soil texture by forming casts or ribbons.
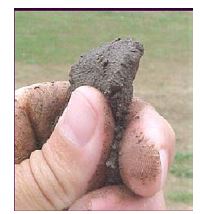
Sand refers to soil that is loose and grainy. The individual grains can be seen or readily felt. When the soil is dry and squeezed between the thumb and forefinger, the sandy soil will fall apart when the pressure is released. When the soil is moist and squeezed in the hand, it forms a cast or ribbon but crumbles when touched.
Sandy loam refers to soil containing a high percentage of sand but having enough silt and clay to make it somewhat coherent. The individual sand grains can be readily seen and felt. Squeezing dry sandy loam soils will form a cast that falls apart readily. If squeezed when moist, a cast can be formed that requires careful handling to prevent it from breaking.
Loam refers to soil having a relatively even mixture of different grades of sand, silt and clay. It has a somewhat gritty feel, but is fairly smooth and slightly flexible. When creating a ribbon with dry loam soil, it forms a cast that holds together with careful handling; but when the ribbon is formed by squeezing moist soil, it can be handled freely without breaking.
Silt loam refers to soil having a moderate amount of fine sand and only a small amount of clay; over half of the particles are of the size called silt. When dry, a silt loam appears cloddy, but the lumps can be broken readily. When pulverized, it feels soft and floury. When wet, the soil runs together readily and puddles.
Either dry or moist, silt loam forms a cast that can be handled freely without breaking; when moistened and squeezed between thumb and finger, it does not form a ribbon but has a broken appearance.
Clay loam refers to a moderately fine-textured soil that usually breaks into clods or lumps that are hard when dry. When the moist soil is pinched between the thumb and finger, it forms a thin ribbon that breaks readily, barely sustaining its own weight. The moist soil is flexible and forms a cast or ribbon that does not readily crumble but works into a heavy compact mass.
Clay refers to a fine-textured soil that usually forms very hard lumps or clods when dry, but is very pliable and usually sticky when wet. When the moist soil is pinched out between the thumb and finger, it forms a long flexible ribbon. Some clays that are very high in colloids are friable (readily crumbled, brittle) and lack plasticity at all conditions of moisture.
The textural triangle (Figure.3) shows the ranges in the percentages of sand, silt and clay associated with the different textural classes.
There are large differences in size between sand, silt and clay particles (Figure 4). The cumulative surface area of soil particles determines the potential amount of available water and plant nutrients that a soil can hold. Clay particles, though very small, have a very large surface area in proportion to their size, and can hold more water and nutrients than a sandy soil.
Light soils (sandy) are often referred to as having a coarse texture, and heavy soils (clayey) as having a fine texture. Light soils are easier to work, contain more air, hold less moisture, have greater leaching potential, contain less organic matter and have greater temperature fluctuation between night and day.
Heavy soils are harder to work and they contain less air, but they hold more moisture, have lower leaching potential, more biological activity and less temperature fluctuation between night and day. Soil structure, organic matter, aeration, bulk density, tilth, water movement and storage, weathering of minerals, and nutrient supply are all influenced, directly or indirectly, by soil texture (Schulte, et al., 2005).
Figure 3. Soil textural triangle.
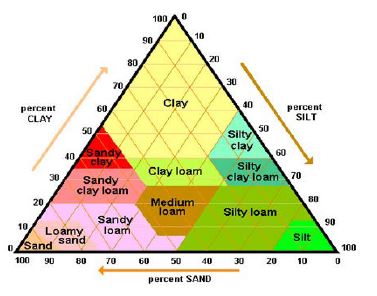
Figure 4. Relative soil particle sizes.
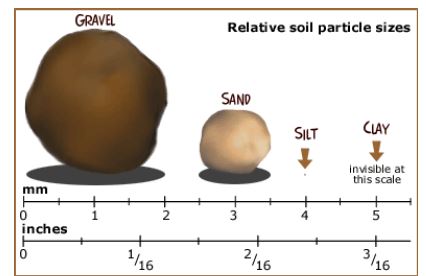
Soil Structure
In all soils except sands, soil particles tend to stick together or aggregate. The arrangement of soil particles into aggregates of definite shape is known as soil structure. Soil structures are also known as peds (Natural Resources Conservation Service, 1991). Peds persist through cycles of wetting and drying. Soil structure is important because it affects water movement into and through the soil, root penetration, porosity or aeration, and bulk density of the soil.
Soil texture has strong influences on soil aggregation. A well-aggregated soil improves soil aeration, water penetration, root growth, nutrient assimilation and release of gasses from the soil. Single-grained and massive soils have no structure. In single-grained soils, such as loose sand, water infiltrates into and percolates through very rapidly. Water moves very slowly through massive soils, such as some clays. Prismatic, blocky and granular structures allow for greater movement of water, while a platy structure impedes the downward movement of water.
Types of soil structures (Figure 5) are:
- Prismatic – aggregates that are tall and narrow, similar to a quartz prism. This structure is found in young soils or in dry/arid regions.
- Blocky – aggregates with sides that are more or less equal. Block-like structure is usually found deep in the soil horizon (profile).
- Platy – aggregates that are much longer (wider) than tall (like a dinner plate). This structure occurs on or near the soil surface and is caused by water pooling or impact from rain
- (crusting).
- Granular – aggregates that are more or less rounded, granular and crumb-like. This structure is found under grass stands near the surface (Natural Resources Conservation Service, 1991).
Unlike texture, the soil’s structure can be changed dramatically to the depth of tillage. Restoring good soil structure involves growing crops with large intensive root systems (e.g., grass and/or legume crops); incorporating livestock manure, crop residue or other organic matter into the soil; controlling erosion; and minimizing raindrop impact on bareground and repeated traffic with heavy equipment across the field. Cycles of wetting and drying, or of freezing and thawing, also improve soil structure. Irrigation water that contains large amounts of sodium causes very undesirable structure by separating the soil aggregates (Natural Resources Conservation Service, 1991).
Figure 5. Examples of soil structure with pore space.
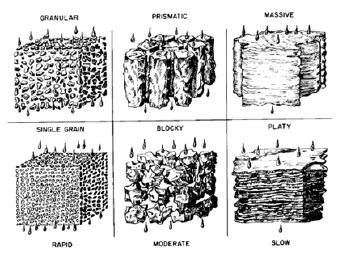
Soil Tilth
Soil tilth is a description of the physical condition of the soil in relation to plant growth, ease of tillage and fitness as a seedbed. It depends on both the degree and stability of soil aggregates. Good, fair and poor are the common descriptive terms for tilth. They refer to the ease with which a soil can be tilled and the rate it takes in water. Good soil tilth can be achieved on most soils by using good soil management practices, such as: reducing or modifying tillage, crop rotation, growing cover crops, adding organic amendments and adding chemical amendments.
Soil Porosity/Bulk Density and Pore Space
In a typical soil, there will be about 50 to 60 percent solid material and 40 to 50 percent pore space, or gaps between the particles (Figure 6). In an ideal soil, about half of the pore space is filled with water and half with air. Pore space is essential for plant growth, providing space for plant roots to grow. Soil pore space also provides a home for living organisms, many of which remove nutrients from organic matter and make them available to the crop. Pore space in soils can be viewed as a vast interconnecting network of voids extending in all directions. Coarse-textured soils tend to be less porous than fine textured soils, but the average size of individual pores is usually larger in sandy soils.
Figure 6. Pore space in sandy soil vs. clay soil.
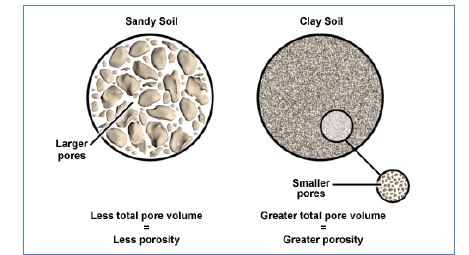
Soil Compaction
Compaction of soils reduces air space and porosity of the soil. The air space may be reduced to as little as 5 percent, reducing the soil’s ability to support plant growth (Figure 7).
Agricultural soils typically become compacted because wheels, tracks or other heavy equipment are applied to the soil surface during cultural operations, particularly when the soil is wet. Trampling by livestock can also cause soil compaction. In an agricultural sense, soils are considered to be compact when movement of air through the soil structure is restricted, which impedes root penetration and water drainage, and often reduces crop production.
Soil Quality
The quality of soil affects all agronomic practices, including which plants will grow on the site, what the optimum irrigation rate should be, and which fertilizers or soil amendments are required. A visual assessment of soil quality is a good first step in determining soil quality and characteristics.
Figure 7. Compacted soil has smaller pore spaces than non-compacted soil.
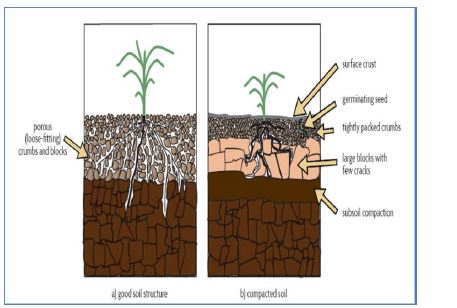
However, biological and chemical characteristics of the soil often require sampling for laboratory testing. In addition to the physical characteristics of the soil, both biological and chemical characteristics can have significant impacts upon plant health, growth and productivity. Understanding soil’s physical characteristics allows a farmer to become a better manager of a more economical and profitable operation.
Works Cited
- Gatzke, H., Gatzke, J., & Holloway, D. L. (2008). Youth Land and Home-site Judging in Nevada, CM 08-08. Reno, NV: UNCE.
- Houghton Mifflin Company. (2005). The American Heritage Science Dictionary. Houghton Mifflin Company.
- McCormick, J. A., & Naphan, E. A. (1955, February). Experiment Station Circular No. 8. Understanding The Irrigated Soils of Nevada.
- Reno, Nevada: University of Nevada Max C. Fleischmann College of Agriculture Agricultural Experiment Station and U.S. Department of Agriculture Soil Conservation Service.
- McDole, R. E., McMaster, G. M., & Larson, D. C. (1974). Available Water-Holding Capacities of Soils in Southern Idaho. CIS 236. University of Idaho Cooperative Extension System and Agricultural Experiment Station.
- National Soil Survey Center. (1996, April). Soil Quality Indicators: Aggregate Stability. Soil Quality Information Sheet. U.S.D.A. Natural Resources Conservation Service.
- Natural Resources Conservation Service. (1991). Section 15, Irrigation. In National Engineering Handbook (2nd ed.). United States Department of Agriculture.
- Natural Resources Conservation Service. (n.d.). Web Soil Survey. Retrieved July 2011, from Web Soil Survey: http://websoilsurvey.nrcs.usda.gov/
- Neufeld, J. (2011). Irrigation Management for Irrigated Pastures. In Intermountain Pasture and Hay Meadow Handbook: Pasture, Hay and Profit EB-00-02 (p. Ch. 4). Reno: UNCE.
- Schulte, E. E., Walsh, L. M., Kelling, K. A., Bundy, L. G., Bland, W. L., Wolkowski, J. B., et al. (2005). Management of Wisconsin Soils, Publication A3588 (5th ed.). Madison, WI: Cooperative Extension Publishing, University of Wisconsin.
- Soil Conservation Service. (1993). National Soil Survey Handbook. U.S. Department of Agriculture.
- Figure 2. Determining Soil Texture by Forming Casts or Ribbons. Michigan Forests Forever Teachers Guide, Michigan State University Extension, soil texture
- Figure 3. Soil Textural Triangle, Wayne Newbill, Soil Texture Triangle of the USDA System
- Figure 4. Relative soil particle sizes, Discovery Education: free teacher resources
- Figure 5. Examples of soil structure with pore space, Managing Soil Tilth: Texture, Structure and Pore Space, Colorado State University, Line drawing from (USDA)
- Figure 6. Pore Space in Sandy Soil vs. Clay Soil, Understanding the Hydrologic Cycle, Understanding the Hydrologic Cycle international Edition
- Figure 7. Compacted soil has smaller pore spaces than non-compacted soil. From Magdoff and Van Es. 2010. Building Soils for Better Crops: Sustainable Soil Management.. SARE Program. Waldorf MD. 294 p.